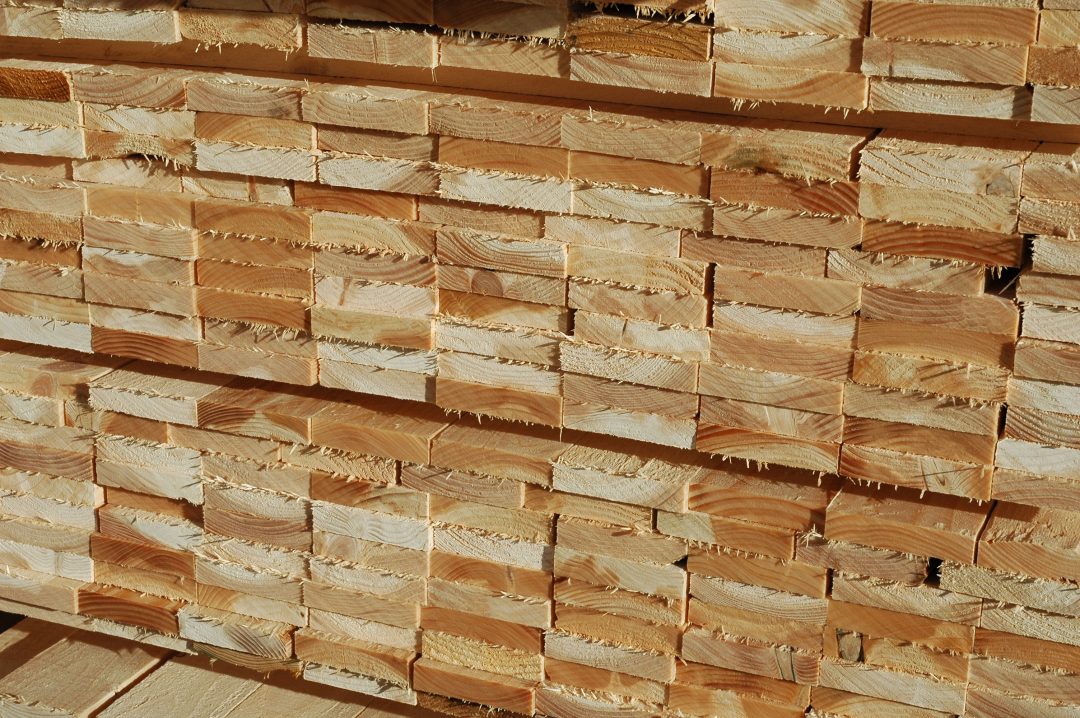
This is a guest post by Dr Morwenna Spear.
Dr Spear is a materials scientist at the BioComposites Centre at Bangor University, specialising in timber. Much of her research relates to new treatments and processes for timber, working with industry to address technical challenges and scale up of new technologies. She also undertakes market studies and trends analysis relating to timber in construction, biobased materials and sustainability.
Timber in construction
The use of wood in construction offers two beneficial effects: displacement of materials with higher embodied carbon, and storage of sequestered carbon dioxide in the built environment for long periods of time. This was demonstrated by Wood in Construction in the UK (WIC) report [1]
[1] Wood in Construction in the UK: an analysis of carbon abatement potential. (2019) https://www.theccc.org.uk/publication/wood-in-construction-in-the-uk-an-analysis-of-carbon-abatement-potential-biocomposites-centre/
There is scope to increase the use of wood in construction in the UK, both through greater adoption of timber framed housing (and related modular construction systems), and through the use of engineered wood systems such as CLT (cross-laminated timber), laminated veneer lumber (LVL) and glulam (glued laminated timber) to deliver larger structures (e.g. apartment blocks) and non-residential structures. There has already been a growth in both systems during the past decade. Some of the increase in uptake of timber framed systems relates to the suitability of the system for delivering the energy efficiency requirements of modern building regulations. Other drivers include speed of delivery and erection on site, factory control, waste minimisation and management of risk.
Engineered wood systems have been shown to deliver high performance buildings, but uptake is lower due to inertia in the construction sector. During stakeholder workshops and interviews (for the WIC report) several barriers were identified, including procurement models and risk management through the sub-contracting and tendering process. Materials consideration and sustainable construction principles are frequently secondary to cost in identifying structural solutions. The recent construction sector initiative for value-based procurement, and integrated processes (e.g. Construction Innovation Hub Value Toolkit) may address some of the underlying inertia through promoting new ways of working and integrated supply chains. Perceived or actual cost of engineered wood structures is sometimes mentioned, however engineers’ or firms’ preferences based on familiarity with other materials (such as steel and concrete), or greater confidence when working with specific material systems, is frequently mentioned by those inside the construction sector.
The trends towards timber framed systems or CLT structures are well aligned with the Construction Sector Deal. This seeks to increase modern methods of construction (MMC) systems and offsite construction to boost UK manufacturing while reducing inefficiency; to shift the focus in procurement away from delivery cost to performance and costs across the building life-cycle; and to deliver reductions in greenhouse gas emissions in the built environment supporting the Clean Growth Strategy.
Timber framed structures and engineered wood structures are increasingly common in the UK, and design principles are well understood. There is however an ongoing need to address perceptions of performance, and raise awareness of the capability of these buildings through case studies and exemplar projects. A large body of examples has been prepared by Wood for Good (https://woodforgood.com/), TRADA and other bodies, which highlight many of the innovations, health benefits, sustainable design principles or aesthetic qualities. The 100 Projects UK CLT book which was published in 2018, gives many examples in different building types (https://info.thinkwood.com/100-uk-clt-projects)
Building performance and areas for action
Additional in-service monitoring and data gathering to support improved knowledge for the new generation of energy efficient buildings is recommended, following on from the successful Building Performance Evaluation project. This is necessary due to the identified challenges surrounding energy efficiency design and the performance gap, and would benefit all structural systems in the quest to refine and improve energy efficient buildings. In addition, the complexities of integrating sustainable technologies such as photovoltaic cells or other renewable energy systems alongside building heating and ventilation controls pose challenges in communicating guidance to occupants, and follow up maintenance. This issue could be addressed in parallel with building performance data-logging and post occupancy assessments.
Skills shortages remain an issue in construction, however the MMCs offer an opportunity to diversify the skills mix required, with a shift towards off-site assembly and manufacturing skills. Ongoing investment in on-site and off-site training is required, including a move to ensure regional access to relevant courses through FE providers.
The MMC systems also offer a significant opportunity for increasing UK manufacturing. All sectors of the supply chain however are at risk of peaks and troughs in the national economy, and it is recommended that consideration is given to some measure which permits growth in capacity to deliver wood in construction systems, while providing security of output even in periods of economic slow-down.
To deliver energy efficient homes in line with the Homes Fit for the Future report (CCC) more work is required. The availability of technologies to deliver this heating to displace the gas grid is currently not in place, although emerging. The challenges of energy efficiency and ventilation within buildings while maintaining indoor air quality are being researched, but sufficient evidence to support mandatory introduction of new designs across the mass house-building sector is unlikely in the timescale indicated. Additional work on this and the performance gap [2] is needed.
[2] Performance gap, i.e. design performance versus in service performance. A related issue is the longevity of this performance, it would be wise to monitor the in-service performance of new systems after 1, 5, 10 and more years.
The timber framed housing starts as a proportion of total housing starts have increased (28% in 2016, Structural Timber Association), however the desire for high levels of timber framed dwellings by 2025 remains a challenge. In stakeholder interviews, companies indicated an interest in rolling out additional capacity but stressed that businesses require confidence in market demand to make the investment and undergo retraining necessary to move from one structural system to another. Good initiatives by Welsh Government including the Home Grown Homes project (https://woodknowledge.wales/home-grown-homes) are supporting substantial growth in the timber frame sector, and importantly have worked with the whole supply chain, from forest upwards.
The other key consideration is the decarbonisation pathway of each material sector represented in construction. Many building materials (glass, ceramics, polymers, metals, in additional to bricks, steel, concrete and timber) are needed for a successful build. Thus the sector relies on initiatives to decarbonise or increase energy efficiency within each materials sector. The progress in some of these sectors is mapped out towards 2050 in decarbonisation strategies, but many foundation industries rely on carbon capture and storage (CCS) or carbon offsetting to achieve their net-zero targets. If this is the case then central support is required to invest in CCS technology, and in future further strategic investment will be required to connect the geographically dispersed emitting industrial sites relating to these unavoidable or ongoing emissions.
Materials choices for lower embodied carbon – environmental product declarations (EPDs)
Considering materials choice to reduce building carbon impact has to be done holistically, i.e. for the whole building design, not simply by substituting one element for another material. The introduction BS EN 15978 makes this technique viable on a designing-for-materials-choice basis, by calculating the embodied carbon for the built unit, for all life cycle stages.
Combining the many necessary building materials in a carbon-efficient, and materials-efficient manner, in addition to ensuring energy efficient design for occupancy stage, is required.
Substantial progress has been made in this area in a relatively short space of time. The standard for calculating embodied carbon of new buildings is BS EN 15978:2011. Guidance to architects, chartered surveyors and structural engineers is now well publicised through professional bodies (RIBA [3], RICS [4], IStructE [5]). It would be relatively straightforward to progress this further through policy measures.
[3] RIBA (2018) Embodied and whole life carbon assessments for architects, https://www.architecture.com/knowledge-and-resources/resources-landing-page/whole-life-carbon-assessment-for-architects
[4] RICS (2017) Whole life carbon assessment for the built environment professional statement, https://www.rics.org/uk/upholding-professional-standards/sector-standards/building-surveying/whole-life-carbon-assessment-for-the-built-environment/
[5] IStructE (2020) How to calculate embodied carbon, https://www.istructe.org/resources/guidance/how-to-calculate-embodied-carbon/
Quantification of stored sequestered carbon within structures is not widely practiced, and guidance in this area is less plentiful and less definitive. Further research and studies by industry are required to establish best practice for how to account carbon release at end of life, and to establish accurate values for current timber recycling, reuse, energy generation for the UK. There must also be a commitment to revise this data periodically as developments occur in the recycling sector; as changes in market activity and demolition reclamation practices occur; and as energy policy (around biomass energy) changes over time.
Emissions relating to occupation of the building, e.g. heating and lighting use, cannot currently be addressed using this LCA-based [6] standard. Many factors must be considered to relate building design (influences such as thermal mass, hygrothermal performance, ventilation, solar gain, use of passive shading systems, phase change materials etc) to the energy demand of the building. This can be done in computer models at design stage, but will still overlook the influence of occupant choices and preferences, familiarity or training of the occupants for new technologies, occupant ability to engage with the energy efficiency installations etc. Initial studies indicate that a substantial body of extra work is required in this field.
[6] LCA = Life Cycle Assessment
Nature-based materials
Several nature-based materials can contribute to reducing the embodied carbon of a building. The use of timber framed systems has been calculated to reduce carbon compared to masonry equivalents. The use of engineered wood systems has been shown in case studies to reduce carbon compared to steel or concrete systems. This means that timber and engineered wood products can contribute to reducing embodied carbon of houses and larger non-residential structures. However, for all construction types reductions can also be made by good design to maximise carbon efficiency of the structure, considering the carbon emissions associated with each product at the design stage and evaluating alternative products prior to build. The use of EPDs as a design tool prior to construction can support this.
Other natural materials, such as sheep’s wool, hemp fibre, recycled cotton fibre, hemp fibre, wood wool insulations, can also substantially reduce the embodied carbon on a per area basis compared to some other insulation products. However, the details of design must be carefully worked out, as thermal conductivity of these natural fibre insulations is in the same ball park as mineral wool, but not as effective as polyurethane foams or aerogels, for example. Design of a total wall assembly using calculated proportions of insulation A and insulation B, considering the thermal properties and embodied carbon together would be required for each installation. The allowable wall thickness may become a limiting parameter, but the desired interior and exterior wall finishes, and any air gap to avoid dew point would also require consideration.
Other wall assemblies such as straw bale with clay, or cob walls including straw or hemp shiv, can also be considered, and may have a niche appeal for some clients and builders. Here large savings of embodied carbon can be achieved, but the UK currently does not have infrastructure to deploy these technologies on large scale. Investment in skills, new business development and suitable certification processes would require consideration if this were adopted going forwards.
A second consideration for this question is the stored sequestered carbon within the biobased materials. This can be substantial; and is already substantial in existing housing stock, even in masonry systems as timber is widely used for flooring and roofing of many houses. This was covered in the WIC report to the CCC (2019), and other studies have looked in greater detail recently at larger structures [7].
[7] e.g. Hawkins et al. (2021) Embodied carbon assessment using a dynamic climate model, Structures 33:90-98
The stored sequestered carbon is counted separately from the embodied carbon associated with the building elements. The options for reclaiming timber at end of life, for re-use in structures, or for recycling into new products such as chipboard, further extends the benefit of carbon storage beyond the building service life. Caution is required to avoid over-simplification (e.g. assuming all timber is burnt or landfilled at end of life), and as demonstrated by Hawkins[7], even use of demolition timber in bioenergy can have a beneficial effect compared to wasteful use of the timber. The current industry practice of reclaimed timber being recycled into chipboard and similar products [8] is also beneficial. More work is required in this area to provide worked examples which reflect the high levels of wood recycling in the industry in the carbon storage data.
[8] In 2019 984 thousand tonnes of recycled and reclaimed wood was used in UK manufacture of wood based panels. This was 25% of resource intake by panel mills.
However, even in simple examples that assume timber is instantly oxidised on removal from the structure at end of life, have demonstrated a substantial benefit. For a national scale study using UK house building and demolition data, a flux of stored sequestered carbon into housing stock was 1.1 to 1.2 MtCO2e p.a. was calculated [9]. This study demonstrated a stocks and flows approach to UK building stock as a store of sequestered carbon. Where the flows into the building products pool exceed the flows out from the pool, the quantity of sequestered carbon stored within the pool increases. Current housebuilding greatly exceeds demolitions, thus storage of sequestered carbon is occurring.
[9] Spear et al. (2020) A conceptual methodology for estimating stored sequestered carbon in the built environment, in: Smart Innovation, Systems and Technologies, Vol 23, Sustainability and Energy in Buildings 2020, Littlewood et al. (Eds.), pp381-392
What are other nations doing?
A review of policies from other regions reveals that both wood content and embodied carbon of new buildings have been used within legislation and policy to deliver sustainability objectives in construction.
Wood based policies include: Wood first (British Columbia, Canada); Wood Charter (Quebec, Canada); Grenelle I – timber ratio within buildings (France); Wood Resource Policy – timber per building (Switzerland)
Embodied carbon or lifecycle costing or Lifecycle analysis policies include: Procurement policy (Government of Canada); Procurement Policy – Buying Green: a handbook on public procurement (European Commission); Netherlands Building Decree – embodied carbon of buildings (The Netherlands); Assessment System for Sustainable Building certification – whole building LCA (Germany); Re-zoning policy – whole building LCA (City of Vancouver, BC, Canada); Carbon footprint limits for building types (Finland).
The recent rapid increase in total numbers of Environmental Product Declarations (EPDs), including many for construction products, means that carbon calculations for buildings (or embodied carbon, or global warming potential, GWP) can be performed with increasing ease and accuracy. Several bodies, including RIBA, RICS and IStructE, have offered solid guidance to their members and wider construction community about the processes required for EPDs at building level. There is also a British Standard relating to the process (BS EN 15978:2011).
Leave a Reply
You must be logged in to post a comment.